In Situ Measurments of Acosutic Target
Strengths of Siphonophores
J.D. Warren (1), T.K. Stanton (1),
M.C. Benfield (2), P.H. Wiebe (1), D. Chu (1)
(1)Woods Hole Oceanographic Institution,
Woods Hole MA 02543 USA
email: jwarren@whoi.edu, tstanton@whoi.edu,
pwiebe@whoi.edu, dchu@whoi.edu
(2)Louisiana State University, Coastal
Fisheries Institute, 218 Wetland Resources,
Baton Rouge LA 70803 USA
email: mbenfie@lsu.edu
Measurements
were made of the acoustic target strengths of siphonophores swimming
freely in the ocean. The measurements were made possible by use of a
remotely operated vehicle (ROV) on which both acoustics and video
camera equipment were mounted. The acoustic transducers and camera
were aimed at the same volume of water and data from the two sets of
devices were co-registered. The cameras were used to help search for
and identify the animals and to direct the path of the ROV; so that
once the animals were found they would be in or near the acoustic
beams. The data show that these gas-bearing zooplankton have
relatively high target strengths due to the presence of the gas
inclusion. These data are essential in the use of acoustics for
quantitatively surveying zooplankton for two reasons: 1) It suggests
that a relatively small number of siphonophores can dominate the echo
in an acoustic survey while other species present may dominate the
biomass. 2) Siphonophores are very fragile and, as a result, cannot
be reliably captured by use of nets. These data can help in making
acoustics a quantitative survey tool for these important animals.
1.
Introduction
Acoustics
provides a means by which marine life such as zooplankton and fish
can be rapidly surveyed within a large body of water [Medwin and
Clay, 1998]. Knowledge of the target strengths of the animals is
critical to the interpretation of acoustic survey data. Target
strength information relates the echoes from acoustic surveys to
meaningful biological parameters such as length and numerical
density. Because of the complexity of the shape and material
properties of various marine life, scattering data are required to
formulate an accurate model for target strength. Because of the
logistical constraints of performing controlled measurements at sea,
most scattering measurements have been done in a laboratory.
Although laboratory measurements can be highly controlled, there are
also artifacts associated with them such as the animal being placed
in a shallow tank of water instead of at its natural depth as well as
its desired temperature. Thus there is a great need for making
controlled measurements of target strength in the natural
environment.
One
important component of the zooplankton are the physonect
siphonophores (Fig. 1). They play an important role in the food
chain-- for example, they are predators of copepods, also important
animals [Mackie et al., 1987]. Studies of the distributions
of siphonophores have been limited, to date, by the inability to
sample the animals by the use of nets or pumps. The animals are so
fragile that they are destroyed by most mechanical means of capture
and thus are greatly undersampled. Further, these colonial organisms
are competent swimmers capable of evading slow moving nets. Since
the animals have a gas inclusion (pneumatophore), they can be
detected by use of acoustics. In fact, they are thought to be
potentially significant sources of scattering in the ocean [Barham,
1963]. However, the target strength models of siphonophores, to
date, are based upon scattering data collected in a laboratory tank
where the animals were in 1 meter of water and subsequently at 1 atm
of pressure [Stanton et al., 1998a,b]. Since the gas could,
in principle, shrink with increasing depth, the target strengths
collected at 1 meter of water may not necessarily correspond to what
exists at deeper depths.
Fig.
1. Drawing of a siphonophore. The animal consists mostly of
gelatinous tissue with the exception of a gas inclusion at the top
(pneumataphore). This gas inclusion can be a significant source of
acoustic scattering.
For
the reasons given above, acoustical methods are vital to observing
distributions of siphonophores and because of the gas inclusions,
target strength measurements should be performed in situ as
much as possible.
The
purpose of this presentation is to describe recent in situ
measurements of acoustic target strengths of various siphonophores.
The results are discussed in terms of the ability to use sound to
quantitatively survey siphonophores.
2.
Experiments
The
measurements were performed on the RV SEA DIVER using a MAXROVER ROV.
The experiments were performed off of Cape Cod, Massachusetts, USA
in July, 1998. Abundant layers of siphonophores were observed in
this region.
Both
video cameras and acoustic transducers (24, 120, and 200 kHz) were
mounted onto the front of the ROV and aimed at the same volume of
water. The focal point was 1 m in front of the apparatus. The
acoustic system was calibrated in the range of depths over which the
measurements were performed. Cables from the equipment were tied to
the ROV cable and run up into a shipboard laboratory where the video
images and acoustic echoes could be observed and recorded. Because
of noise transmitted from the ROV cable to the acoustic cables, only
the 24 and 120 kHz systems could provide high quality signals.
During deployment, the ROV was initially aimed into the current so as
to observe marine life swimming or drifting by it. Once an animal of
interest was detected with the camera, the operator would then let
the ROV drift with the animal while rotating the ROV so as to keep
the camera and acoustic beam aimed at the animal. By using this
technique, up to one hundred acoustic pings per animal could be
recorded.
3.
Results
Throughout
the several days of measurements, there were many siphonophores
observed by the acoustic/video system. The echoes from the
siphonophores were generally quite strong (Fig. 2). The echoes were
also variable from ping to ping. This variability was due, in part,
to the fact that the animals changed location within the beam during
the measurements (in spite of efforts by the ROV operator to keep the
ROV positioned and aimed so that the animal would remain in the
center of the beam). Thus the beam pattern was convolved with the
scattering amplitude of the animal.
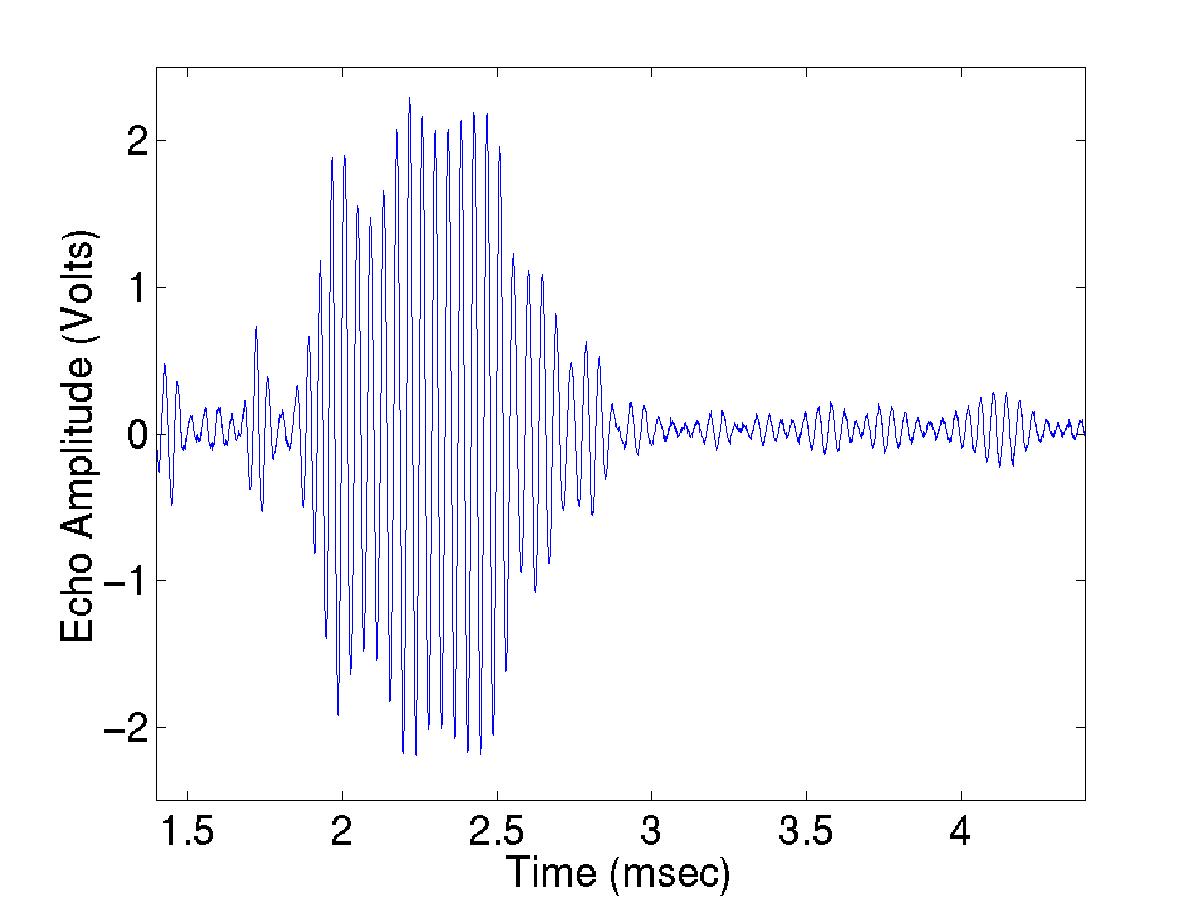
Fig.
2. Echo from a single siphonophore (24 kHz).
In
order to remove the effects of the beam pattern, histograms of the
echo amplitudes (once calibrated and adjusted for range from
transducer) were compared with classes of curves based on
convolution-based echo predictions [Clay, 1983]. These predictions
of echo amplitude histograms convolve the scattering response from a
target with the beam pattern response. Since the gas inclusion is
assumed to be the part of the siphonophore that dominates the
scattering and is also much smaller than an acoustic wavelength, the
scattering amplitude (echo less beam pattern effects) is assumed to
be constant from ping to ping (i.e., no orientation effects). There
are no other assumptions on the scattering properties of the animal
besides this one. By varying the target strength from prediction to
prediction, a class of curves was generated, with one representing
the best fit to the data. The target strength associated with the
best-fit prediction is then the estimate for the target strength of
the animal.
Through
this fitting method, the target strength of the siphonophores was
approximately -60 dB at 24 kHz. This value is higher than values of
target strength measured in the laboratory at higher frequencies.
This increase can be attributed to the fact that at these lower
frequencies, the resonance frequency of the gas is being approached.
For gas bubbles, it is well known that the scattering levels increase
as the frequency approaches resonance.
Another
important observation is the fact that the scattering levels are
consistent with a gas inclusion of about 1 mm, a diameter observed at
the surface from animals captured by nets. This strongly suggests
that the gas of these animals did not significantly shrink at these
deeper depths of the experiment, although the experiments were
limited to ROV depths of about 25-30 m.
4.
Summary and Conclusions
By
use of co-located, co-registered acoustic and video instrumentation
mounted on an ROV, target strengths of individual siphonophores were
measured. The target strengths were relatively high compared with
other zooplankton because of the gas inclusion contained by the
siphonophore.
These
results are important for two reasons: 1) These in situ
measurements confirm earlier reports (from laboratory measurements)
of the high target strengths of siphonophores; and 2) The results can
be used for interpreting acoustic surveys of siphonophores. Because
of the fragile nature of the siphonophores, acoustics is one of the
only viable means of quantitatively surveying the animals.
5.
Acknowledgements
The
authors are grateful to the captain and crew of the RV SEA DIVER for
their skilled efforts on this cruise. The authors also gratefully
acknowledge Ellen Bailey of the Woods Hole Oceanographic Institution
(WHOI) for assistance in the preparation of this manuscript and Dave
Gray (WHOI) for assistance on the video images. This is Woods Hole
Oceanographic Institution contribution number 9924.
Reference
E.G.
Barham, Siphonophores and the deep scattering layer, Science 140, pp.
826-828, (1963).
C.S.
Clay, Deconvolution of the fish scattering PDF from the echo PDF for
a single transducer sonar, J. Acoust. Soc. Am. 73, pp. 1989-1994,
(1983).
G.O.
Mackie, P.R. Pugh, and J.E. Purcell, Siphonophore biology, Advances
in Marine biology, 24, pp. 98-262, (1987).
H.
Medwin, and C.S. Clay, Fundamentals of Acoustical Oceanography,
Boston: Academic Press,1998.
T.K.
Stanton, D. Chu, P.H. Wiebe, L.V. Martin, and R.L. Eastwood, Sound
scattering by several zooplankton groups. I. Experimental
determination of dominant scattering mechanisms, J. Acoust. Soc. Am.
103, pp. 225-235, (1998a).
T.K.
Stanton, D. Chu, and P.H. Wiebe, Sound scattering by several
zooplankton groups. II. Scattering models, J. Acoust. Soc. Am. 103,
pp. 236-253, (1998b).
|